Hypotheses of the Origin of the Image on the Shroud
Wojciech Kucewicz
AGH University of Science and Technology, Kraków, Poland
Jakub S. Prauzner-Bechcicki
Jagiellonian University in Krakow, Poland
The first photograph of the Shroud taken by →Secondo Pia in 1898 is a turning point for hypotheses explaining the origin of the image on it. Prior to it, there were two different approaches: one that referred to the image as an acheiropit (Old Greek: ἀχειροποίητος—‘not made by hands’), and another that assumed it to be the work of an artist. This second hypothesis was put forward by Peter d’Arcis, an Ordinary of the Diocese of Troyes in the 14th century, who sent it to Pope Clement VII (Waliszewski 1987, p. 35) and it is his opinion that heavily influences the study of the Shroud to this day. Although the features of the image visible in the photograph taken by S. Pia lead many researchers to reject this hypothesis, and although the extensive research carried out 80 years later by the Shroud of Turin Research Project (→STURP) team seems to settle the matter definitively (Jumper et al. 1984), there is no shortage of staunch supporters of the thesis that the Shroud is a medieval forgery. Based on contemporary scientific literature, the main hypotheses of the image’s origin can be divided into three categories (Fanti 2014; Fazio 2020):
- the one that assumes a supernatural source for the image,
- the one that assumes natural processes at play, and
- the one that assumes that it is the work of an artist.
The first photograph of the Shroud led to various hypotheses that were formulated at the beginning of the 20th century explaining the creation of the image of the figure. The explanations need to be compared with the original. There are 24 physical and chemical characteristics of the image visible on the Shroud; a hypothesis can only be verified if it can reproduce those characteristics forward (Fanti 2014).
Initially, knowledge of the characteristic properties of the image from the Shroud expanded on a macro scale; only after the research carried out by the STURP team did it also expand on a micro level. Fluorescence measurements using ultraviolet and X-rays and microchemical studies ruled out the possibility of a painted image on the Shroud (Morris, Schwalbe, London 1980; Miller, Pellicori 1981). The image of the figure showed negligible fluorescence, while much higher fluorescence was observed in areas of charring created during the fire of 1532. Computer analysis confirmed that the image contains unique, three-dimensional information (Jumper 1982; Jackson, Jumper, Ercoline 1982; Jackson, Jumper, Ercoline 1984). The image was formed not only in areas of direct contact between the body and the cloth, but also in areas where there was no such contact, such as between the cheeks and nose (Jumper et al. 1984; Basso, Fanti 2007; Fanti et al. 2010a; Fanti et al. 2010b; Fanti 2014). The image does not appear underneath the bloodstains, indicating that the traces of blood must have been created first and only then the image of the figure. The resulting image did not obliterate these traces in the least (Jumper et al. 1984; Fanti et al. 2010a; Fanti et al. 2010b; Fanti et al. 2005; Fanti 2014).
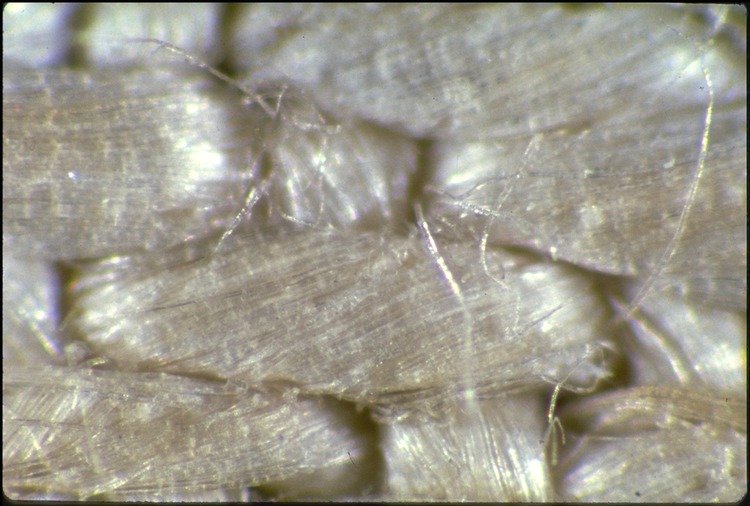
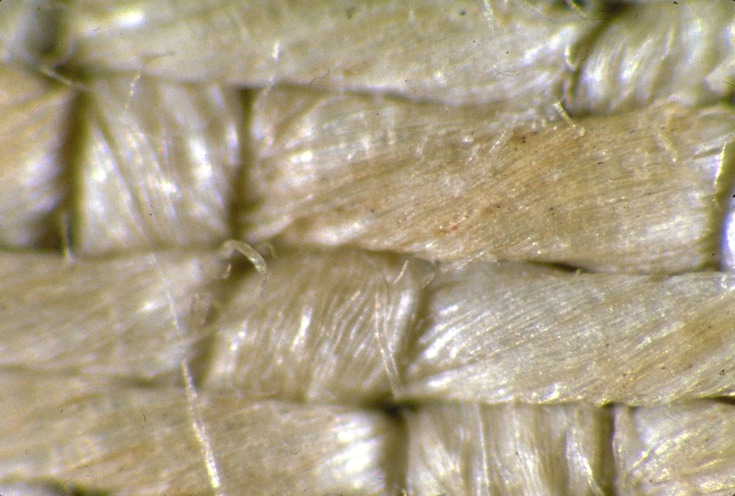
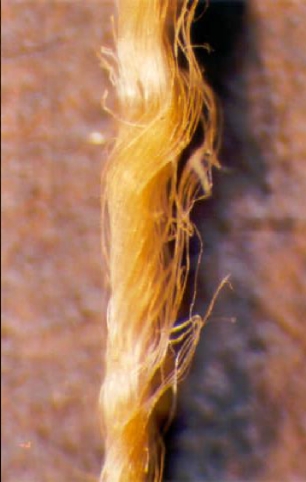
The macro-visible image of the figure was created on fabric woven from threads a fraction of a millimetre in diameter. The threads, in turn, were formed from dozens of linen fibres twisted together. The research showed that in the area of the image, only part of the fibres making up the thread are dyed, while the rest is natural in colour. The differences in the intensity of the image depend on the proportion of yellowed to natural fibres. Where there are more yellowed fibres, the image is darker, and where there are fewer, the image is lighter (Jumper et al. 1984; Fanti et al. 2010a; Fanti et al. 2010b; Fanti et al. 2005; Fanti 2014).
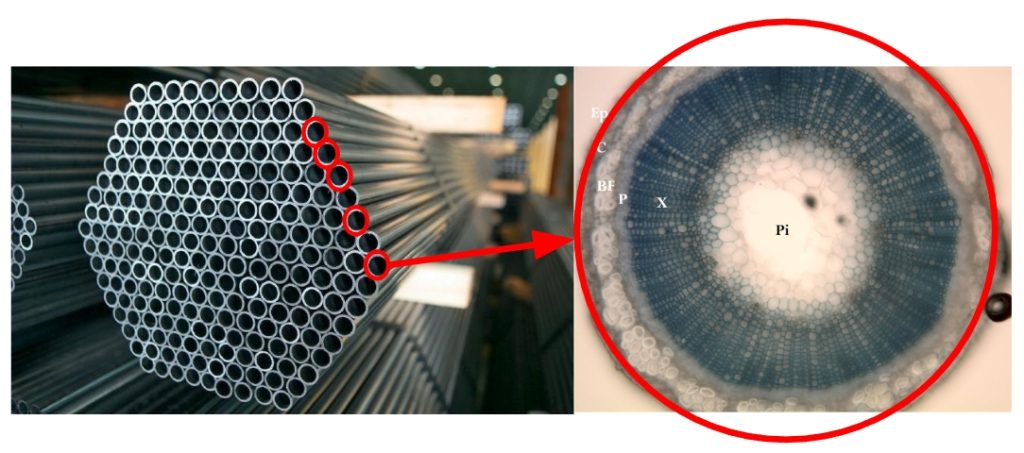
Only the surface layers of the fibres are stained to a small depth (approx. 0.2 μm), i.e. the staining appears only in the outer cells of the fibres (Jumper et al. 1984; Fanti et al. 2010a; Fanti et al. 2010b; Fanti et al. 2005; Fanti 2014). Studies carried out as part of the STURP project lead to the conclusion that this is the result of chemical changes to the structure of cellulose, the basic component of flax fibres (Jumper et al. 1984; Di Lazzaro et al. 2011). The result is the formation of chemical bonds that absorb light from the blue range. The deficiency of this radiation in the visible light spectrum causes the reflected light to be yellow. This phenomenon occurs during the yellowing of paper, which is as a result of cellulose ageing. The rate of ageing depends on the type and amount of energy supplied, which induces structural changes in the cellulose, as well as the presence of a catalyst. The energy could be supplied in the form of heat, light or other radiation.
The catalyst could be substances formed by natural phenomena occurring when a dead body comes into contact with linen cloth. The hypothesis that the image was produced by natural vapours (the phenomenon of vaporography) was put forward by Paul Vignon, and was later developed and modified by other scholars (Waliszewski 1987, p. 112; Rogers, Arnoldi, Review; Rogers 2008). However, the images obtained from the experiments were very different from the image on the Shroud. The shapes were blurred, had poor resolution and, most importantly, the images had no three-dimensional or surface properties (Fanti 2014).
Attempts to obtain the images by providing heat energy (e.g. using a heated relief) were also unsuccessful (Pesce Delfino 2000; Fanti 2014). The resulting images did not have the macroscopic properties that the Shroud has (Fanti 2014), and when subjected to heat radiation the fabric fibres stained beyond the surface. For thermal energy to cause only superficial changes in the structure of the fibres, it should act for a fraction of a second, which is unattainable. The use of ionising radiation (e.g. X-rays or gamma rays) is also out of the question, as it has high energy. It would induce structural changes in the entire volume of the fibres, which is not the case with the image from the Shroud.
Promising analyses using laser radiation in the ultraviolet range were carried out between 2005 and 2010 by a team of researchers led by Paolo Di Lazzaro at the ENEA Research Centre in Frascati in Italy (Di Lazzaro et al. 2010; Di Lazzaro et al. 2011). By irradiating the linen fabric with pulsed laser radiation, with pulses lasting a fraction of a second, an attempt was then made to obtain, at room temperature, colours similar to those observed in the image from the Shroud1. Specifically, the surface staining of the flax fibres was targeted. The best results were obtained using the ArF laser, which emits radiation at 193 nm (Di Lazzaro et al. 2010). By selecting the power density appropriately, controlled by the number of laser pulses, it was possible to obtain staining with fluorescence properties similar to those on the Shroud at a power density of 200 MW/cm2. It was observed that the outer layer of the threads is stained. The colour of the fibres comprising the thread was also analysed and in several cases a surface staining like that on the Shroud was detected.
The researchers also estimated the power of the pulse of radiation that would be needed to produce an image of a figure the size of the one on the Shroud: to do so would require 34 million MW (Di Lazzaro et al. 2011), which, even with modern technological developments, is unfeasible.
Some researchers favour the hypothesis that the image on the Shroud may have been produced by a corona discharge (Fanti, Lattarulo, Scheuermann 2005; Fanti 2010; Fanti 2014), also known as incomplete discharge. This is caused by the ionisation of a gas (e.g. air) and the formation of a plasma in the area between two electrodes with a high potential difference, which is, however, lower than the breakdown voltage, but sufficient to produce an avalanche effect. The ionised gas and the resulting electrons are the source of the current flowing between the electrodes. At the same time, ion recombination can be observed, resulting in the emission of blue-white light with a high ultraviolet content. With asymmetrical electrodes, the corona radiation phenomenon will also be non-uniform. It will be more intense in areas where the electrode surfaces are more similar.
Experimental results were presented in 2005, which confirmed that the image could arise from a corona discharge (Fanti, Lattarulo, Scheuermann 2005). Research in this direction is being carried out by →Giulio Fanti of the University of Padua (Fanti 2010; Fanti 2014). The images obtained on the linen cloth had similar properties to the image on the Shroud, including surface character and three-dimensional information (Fanti 2014).
There are researchers who take the distribution of the yellowed fibres as a starting point. This is the so-called stochastic hypothesis (Fazio, Mandaglio 2011). Its authors, taking as their starting point the observation that the intensity of the image is a linear function of the distance between the cloth and the body wrapped in it (Jackson, Jumper, Ercoline 1984), suggest that the same relationship describes the amount of energy absorbed per unit area, and thus that it also describes the probability of the yellowing of the fibres (Fazio, Mandaglio 2011). They therefore claim (Fazio, Mandaglio 2011; Fazio, Mandaglio 2012), that their hypothesis is consistent with the claims of STURP researchers about the latent nature of the image, i.e. that the image became visible over time due to cellulose degradation (Pellicori, Evans 1981; Schwalbe, Rogers 1982). The time at which an image can become visible depends on the underlying cause: an image can become visible after a few hours, after many years or after decades (Fazio, Mandaglio, Anastasi 2017; Fazio, Mandaglio, Anastasi 2019).
The various hypotheses explaining the origin of the image on the Shroud provoke lively polemics between their authors (Fazio et al. 2015; Fanti 2015; Fazio, Mandaglio 2015). From a scientific point of view, this is a very good situation, providing an opportunity to develop a correct conception.
1 Laser pulses of different lengths were used: 120 ns, 30 ns and 12 ns, where 1 ns (nanosecond) is a billionth of a second. ↑
References
Basso R., Fanti G., Optics Research Applied to the Turin Shroud: Past, Present, Future, [in:] Optics Research Trends, ed. P.V. Gallico, New York 2007, p. 4.
Di Lazzaro P. et al., Deep Ultraviolet Radiation Simulates the Turin Shroud Image, “Journal of Imaging Science and Technology” 2010, Vol. 54(4), pp. 40302-1–6, http://dx.doi.org/10.2352/J.ImagingSci.Technol.2010.54.4.040302.
Di Lazzaro P. et al., Colorazione simil-sindonica di tessuti di lino tramite radiazione nel lontano ultravioletto: riassunto dei risultati ottenuti presso il Centro ENEA di Frascati negli anni 2005-2010, Rapporto Tecnico ENEA-RT-2011-14, [on-line:] https://www.academia.edu/3648806/Colorazione_simil_sindonica_di_tessuti_di_lino_tramite_radiazione_nel_lontano_ultravioletto_riassunto_dei_risultati_ottenuti_presso_il_Centro_ENEA_di_Frascati_negli_anni_2005_2010 – 29 X 2021.
Fanti G., Can Corona Discharge Explain the Body Image of the Turin Shroud?, “Journal of Imaging Science and Technology” 2010, Vol. 54(2), pp. 20508-1-11, http://dx.doi.org/10.2352/J.ImagingSci.Technol.2010.54.2.020508.
Fanti G., Hypotheses Regarding the Formation of the Body Image on the Turin Shroud: A Critical Compendium, “Journal of Imaging Science and Technology” 2014, Vol. 55, No. 6, pp. 60507-1-14, https://doi.org/10.2352/J.ImagingSci.Technol.2011.55.6.060507.
Fanti G., Comments on a Stochastic Hypothesis about the Body Image Formation of Turin Shroud, “The Journal of The Textile Institute” 2015, Vol. 106, No. 8, pp. 900–903, https://dx.doi.org/10.1080/00405000.2014.961265.
Fanti G., Lattarulo F., Scheuermann O., Body Image Formation Hypotheses Based on Corona Discharges, [in:] The Third Dallas International Conference on the Shroud of Turin, Dallas 8-11 September 2005, [on-line:] http://sindone.dii.unipd.it/giulio.fanti/research/Sindone/corona.pdf – 29 X 2021.
Fanti G. et al., Evidences for Testing Hypotheses about the Body Image Formation of the Turin Shroud, [in:] The Third Dallas International Conference on the Shroud of Turin: Dallas, Texas, September 8-11, 2005, [on-line:] http://www.shroud.com/pdfs/doclist.pdf – 29 X 2021.
Fanti G. et al., Microscopic and Macroscopic Characteristics of the Shroud of Turin Image Superficiality, “Journal of Imaging Science and Technology” 2010a, Vol. 54(4), pp. 40201-1-8, http://dx.doi.org/10.2352/J.ImagingSci.Technol.2010.54.4.040201.
Fanti G. et al., List of Evidences of the Turin Shroud, [in:] Proceedings of International Workshop on the Scientific Approach to the Acheiropoietos Images (ENEA, Frascati, Italy, 2010), Frascati 2010b.
Fazio G., The Shroud Body Image Generation: Immanent or Transcendent Action?, “Scientia et Fides” 2020, Vol. 8, No. 1, pp. 33–42, http://dx.doi.org/10.12775/SetF.2020.003.
Fazio G., Mandaglio G., Stochastic Distribution of the Fibrils that Yielded the Shroud of Turin Body Image, “Radiation Effects & Defects in Solids” 2011, Vol. 166, No. 7, pp. 476–479, https://doi.org/10.1080/10420150.2011.566877.
Fazio G., Mandaglio G., Can a Latent Image Explain the Characteristic of the Shroud Body Image?, “Radiation Effects & Defects in Solids” 2012, Vol. 167, No. 3, pp. 220–223, https://dx.doi.org/10.1080/10420150.2011.595413.
Fazio G., Mandaglio G., Commentary: Stochastic Effects and Corona Discharge for the Shroud Body Image Generation, “The Journal of The Textile Institute” 2015, Vol. 106, No. 8, pp. 904–906, http://dx.doi.org/10.1080/00405000.2014.961266.
Fazio G., Mandaglio G., Anastasi A., Revisiting a Pure Stochastic Mechanism to Explain the Body Image Formation on the Linen of Turin, “The Journal of The Textile Institute” 2017, Vol. 108, No. 9, pp. 1552–1555, https://dx.doi.org/10.1080/00405000.2016.1262200.
Fazio G., Mandaglio G., Anastasi A., Describing, Step by Step, the Shroud Body Image Formation, “Heritage” 2019, Vol. 2(1), pp. 34–38, http://dx.doi.org/10.3390/heritage2010003.
Fazio G. et al., Comparison Among the Shroud Body Image Formation Mechanisms by the Linen Fibrils Distributions, “The Journal of The Textile Institute” 2015, Vol. 106, No. 8, pp. 896–899, http://dx.doi.org/10.1080/00405000.2014.930575.
Jackson J.P., Jumper E.J., Ercoline W.R., Three-Dimensional Characteristic of the Shroud Image, [in:] IEEE 1982 Proceedings of the International Conference on Cybernetics and Society, [on-line:] https://www.shroud.com/pdfs/3D%20Characteristic%20Jackson%20Jumper%201982%20OCR.pdf – 20 X 2021.
Jackson J.P., Jumper E.J., Ercoline W.R., Correlation of Image Intensity on the Turin Shroud with the 3-D Structure of a Human Body Shape, “Applied Optics” 1984, Vol. 23, No. 224, pp. 2244–2270, https://doi.org/10.1364/AO.23.002244.
Jumper E.J., An Overview of the Testing Performed by the Shroud of Turin Research Project with a Summary of Results, [in:] IEEE 1982 Proceedings of the International Conference on Cybernetics and Society, October 1982, pp. 535–537.
Jumper E.G. et al., A Comprehensive Examination of the Various Stains and Images on the Shroud of Turin, “Archaeological Chemistry III” 1984, pp. 447–476, ACS Advances in Chemistry, Vol. 205, https://doi.org/10.1021/ba-1984-0205.ch022 with references.
Miller V.D., Pellicori S.F., Ultraviolet Fluorescence Photography of the Shroud of Turin, “Journal of Biological Photography” 1981, Vol. 49, No. 3, pp. 71–85.
Morris R.A., Schwalbe L.A., London J.R., X-Ray Fluorescence Investigation of the Shroud of Turin, “X-Ray Spectrometry” 1980, Vol. 9, No. 2, pp. 40–47, https://doi.org/10.1002/xrs.1300090203.
Pellicori S.F., Evans M.S., The Shroud of Turin Through the Microscope, “Archaeology” 1981, Vol. 34(1), pp. 34–43.
Pesce Delfino V., E l’uomo creò la Sindone, Bari 2000, p. 49.
Rogers R.N., A Chemist’s Perspective on the Shroud of Turin, 2008.
Rogers R.N., Arnoldi A., Scientific Method Applied to the Shroud of Turin, a Review, [on-line:] http://www.shroud.com/pdfs/rogers2.pdf – 29 X 2021.
Schwalbe L.A., Rogers R.N., Physics and Chemistry of the Shroud of Turin: A Summary of the 1978 Investigation, “Analytica Chimica Acta” 1982, Vol. 135, No. 1, pp. 3–49, https://doi.org/10.1016/S0003-2670(01)85263-6.
Waliszewski S., Całun Turyński dzisiaj, Kraków 1987.
Sources of Images
1. Private collection
2. and 3. Sindonology.org, Photomicrographs, http://www.sindonology.org/photomicrographs.shtml
4. Own elaboration of K. Sadło